CF Patients Could Benefit From New Lung Infection Detector Nanodevice, Wearable Tech Prototypes
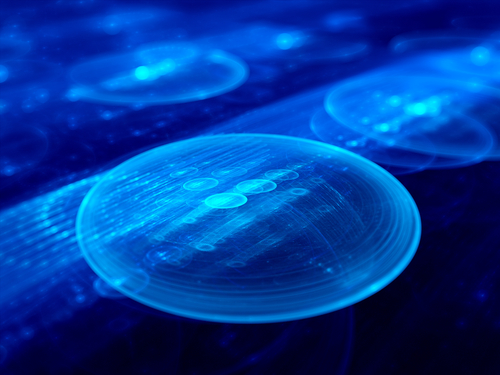
A low-cost, disposable breath analysis device that a person with cystic fibrosis could use at home along with a smartphone to immediately detect a lung infection, much like the device police use to gauge a driver’s blood alcohol level, may soon be a reality.
Such a device could provide timely knowledge of a lung infection and cue people with CF or other inflammatory respiratory conditions to seek immediate treatment, thereby preventing life-shortening permanent damage to already vulnerable airways.
Thanks to a nearly $1.3 million grant from the National Science Foundation, University of California, Irvine (UCI) University of California, Irvine (UCI) engineers will be able to continue developing this type of nanotechnology device and potentially many others using a more wide-scale manufacturing process.
UCI Materials scientist Regina Ragan and electrical engineer Filippo Capolino have created a nano-optical sensor that can detect trace levels of infection in a small sample of breath. They made the sensor as a laboratory experiment, but ideally would like to see it become commercially available. In addition to diagnosing medical conditions, the device could be modified to monitor environmental conditions — for instance, identifying harmful airborne agents produced through automotive or chemical industry practices.
[adrotate group=”1″]
Nanotechnologies such as this lung infection sensor depend on extremely small, nanometer-scale building blocks. A nanometer is about 100,000 times smaller than the width of a human hair. Fabricating on this tiny scale poses huge challenges, since most of the current methods that achieve a high level of precision are too costly and slow to be viable for manufacturing.
“With support from the NSF and input from industry, our goal is to help nanoscale manufacturing processes leave the laboratory where they’ve been confined and become usable in widespread commercial applications,” says Ragan, an associate professor of chemical engineering and materials science and principal investigator on the project, in a UCI release.
“This grant highlights the strength of our faculty in both nanosciences and advanced manufacturing,” says Gregory Washington, dean of The Henry Samueli School of Engineering. “The Samueli School is poised to move forward as a force in this area.”
Dr. Ragan’s research at the Orange County institution involves the exploration and development of novel material systems for nanoscale electronic and optoelectronic devices. One of the key issues in incorporating diverse materials in electronic and optoelectronic devices is to understand the material interfaces and how these affect electronic and/or optical properties. The effect of material interfaces is particularly important with scaling down to nanometer dimensions where the surface to volume ratio is high. Moreover, since the dimensions of devices will reach the dimensions of molecules, she and her research group strive to understand these interfaces at the atomic and molecular level, using self-assembly to fabricate one-dimensional and zero-dimensional organic/inorganic nanostructure arrays. The correlation of material interfaces with electron transport along the lateral axis of nanowires and transport through a molecule/metal nanowire junction is being studied to understand how these components will behave in nanoscale devices.
Dr. Capolino’ Research foci include electromagnetic and optic artificial materials (metamaterials), electromagnetic bandgap (EBG, or photonic bandgap, PBG) materials and plasmonic structures, and their applications in sensors, superlensing, nanotechnology, antennas and waveguides; enhanced radiation and high-directivity antennas (microwaves and optics) with artificial materials, waves in thin films and plasmons; wireless systems at microwaves and sub-millimeter waves. Array antennas and miniaturized antennas; radiation by ultra-wide-band (UWB) pulsed-array antennas; theory and application of electromagnetism in general; short-pulse UWB analysis for imaging and radars; high-frequency field modeling, diffraction and propagation; numerical methods, fast solvers, an hybrid method of moments (MoM)-high frequency analysis.
Co-principal investigators are Dr. Capolino, associate professor of electrical engineering and computer science; Ozdal Boyraz, and Chancellor’s Professor of mechanical and aerospace engineering Marc Madou.
Dr. Boyraz works on integrated optics and optical communications systems. In particular, his recent research activities focus on silicon photonics and nonlinear optics in silicon. He develops active photonic devices in silicon which is compatible with electronic ICs and work on the same platform. Dr. Boyraz is also co-inventor of Silicon Raman lasers, Raman lasers in SiGe alloys, electronic switching of Raman lasing.
Dr. Madou’s UCI BioMEMS laboratory applies miniaturization science to solve chemical and biological problems with an emphasis on molecular biology and energy. The lab group’s research areas include medical diagnostics, sensor technology, micro-battery development, and novel drug delivery systems among others. Currently they are developing several platforms including Microfluidic Compact Discs, Carbon-MEMS devices and Electroactive Polymer substrates, actively pursuing original research through collaborative efforts in academia and industry.
University of Michigan Team Developing Wearable Tech For Disease Monitoring
Meanwhile, a new wearable vapor sensor being developed at the University of Michigan could also one day offer continuous disease monitoring for patients with diabetes, high blood pressure, anemia or lung disease.
Wearable technologies, which include Google Glass and the forthcoming Apple iWatch, are part of a device market that’s expected to swell to $14 billion in value over the next four years.
The U-M sensor, which can detect airborne chemicals either exhaled or released through the skin, would likely be the first wearable to pick up a broad array of chemical, rather than physical, attributes, and researchers are working with the National Science Foundation’s Innovation Corps program to move the device from the lab to the marketplace.
“Each of these diseases has its own biomarkers that the device would be able to sense,” says Xudong (Sherman) Fan, a professor of biomedical engineering. “For diabetes, acetone is a marker, for example.”
Other chemicals it could detect include nitric oxide and oxygen, abnormal levels of which can point to conditions such as high blood pressure, anemia or lung disease.
A paper, entitled “Graphene nanoelectronic heterodyne sensor for rapid and sensitive vapor detection“ (Nature Communications 5, Article number: 4376 doi:10.1038/ncomms5376) recently published in Nature Communications is coauthored by Girish S. Kulkarni, Karthik Reddy and Zhaohui Zhong of the Department of Electrical Engineering and Computer Science, University of Michigan at Ann Arbor; with Karthik Reddy and Xudong Fan of the University of Michigan Department of Biomedical Engineering.
The coauthors note that nearly all existing nanoelectronic sensors are based on charge detection, where molecular binding changes the charge density of the sensor and leads to sensing signal. However, intrinsically slow dynamics of interface-trapped charges and defect-mediated charge-transfer processes significantly limit those sensors’ response to tens to hundreds of seconds, which has long been known as a bottleneck for studying the dynamics of molecule–nanomaterial interaction and for many applications requiring rapid and sensitive response.
In this study, the U-M research team reports a fundamentally different sensing mechanism based on molecular dipole detection enabled by a pioneering graphene nanoelectronic heterodyne sensor, the dipole detection mechanism of which has been confirmed by a range of experiments with vapor molecules of various dipole moments, particularly, with cis- and trans-isomers that have different polarities.
Dr. Fan is developing the sensor with Zhaohui Zhong, an associate professor of electrical and computer engineering, and Girish Kulkarni, a doctoral candidate in electrical engineering. The researchers say their device is faster, smaller and more reliable than its counterparts, which today are much too big to be wearable. The new sensor can also detect a broader array of chemicals.
Beyond disease monitoring, the sensor has other applications. It would be able to register the presence of hazardous chemical leaks in a lab, or elsewhere, or provide data about air quality.
“With our platform technology, we can measure a variety of chemicals at the same time, or modify the device to target specific chemicals. There are limitless possibilities,” Dr. Zhong says in an U-M release.
To create their technology, the researchers took a unique approach to detecting molecules.
“Nanoelectronic sensors typically depend on detecting charge transfer between the sensor and a molecule in air or in solution,” Mr. Kulkarni explains.
However, these previous techniques typically led to strong bonds between the molecules being detected and the sensor itself. That binding leads to slow detection rates.
“Instead of detecting molecular charge, we use a technique called heterodyne mixing, in which we look at the interaction between the dipoles associated with these molecules and the nanosensor at high frequencies,” Dr. Girish says.
This technique, made possible through the use of graphene, results in extremely fast response times of tenths of a second, as opposed to the tens or hundreds of seconds typical in existing technology. It also dramatically increases the device’s sensitivity. The sensor can detect molecules in sample sizes at a ratio of several parts per billion.
These nanoelectronic graphene vapor sensors can be completely embedded in a microgas chromatography system, which is the gold standard for vapor analysis, the researchers say. The entire microgas chromatography system can be integrated on a single chip with low power operation, and embedded in a badge-sized device that can be worn on the body to provide noninvasive and continuous monitoring of specific health conditions.
“We believe this device can be extremely beneficial to society,” says Dr. Fan
Sources:
University of California, Irvine
University of Michigan
Nature Communications
Image Credits:
University of California, Irvine
University of Michigan