“Laser Trapping” Technique Could Lead To Better Management of Bacterial Infections in CF Patients
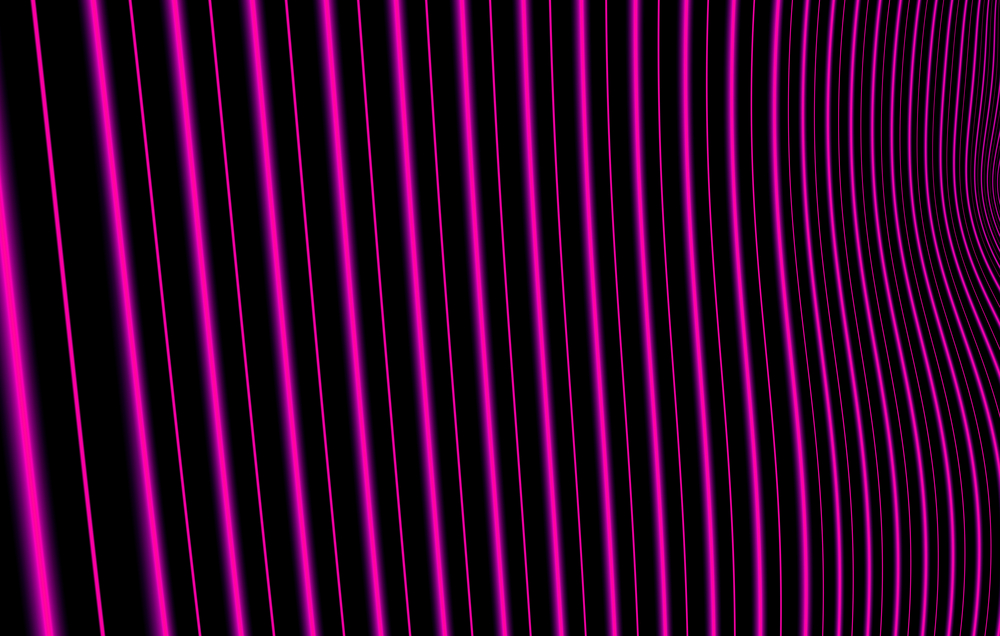
In a report published this week by SPIE — an international not-for-profit society serving more than 256,000 constituents from approximately 155 countries advancing an interdisciplinary approach to the science and application of light — entitled “Laser trapping to show the effects of bacterial arrangement on biofilm infection” (30 December 2014, SPIE DOI: 10.1117/2.1201412.005727) University of Texas at Austin professor at the UTA Department of Physics’ Center for Nonlinear Dynamics Vernita D. Gordon and her Graduate Research Assistant Chris Rodesney explain that Biofilms — sessile (unable to move about) interacting unicellular organism communities that adhere to surfaces and one another — can form on virtually any surface. Examples are biofouling and biocorrosion of industrial equipment, and infections of medical devices and patient tissues in healthcare institutions. The findings could help researchers better understand and treat bacterial infections in Cystic Fibrosis patients, particularly those who are prone to infection from Pseudomonas aeruginosa.
The coauthors note that while traditionally, microbiologists have focused primarily on thoroughly-mixed fluid cultures containing suspended free-swimming cells, most natural settings bacteria are typically found in biofilms that reinforce the bacterial constituents’ resistance to removal efforts and and consequent harm to the host surfaces. Gordon and Rodesney say that understanding biofilms’ properties can facilitate identification of biofilm agents of the worst clinical outcomes by identification of targets for diagnostics and treatment.
The scientists observe that there is broad consensus that the spatial structure of a biofilm is a key factor in its persistence, controlling transport of nutrients, oxygen, and other chemical activity. Moreover, they explain that a biofilm’s structure defines intercellular interaction zones which can modify metabolism and signaling profiles that for example can increase antibiotic resistance and host-damaging virulence factor production.
Dr. Gordon and Mr. Rodesney observe that mechanisms by which spatial structures affect the development and progress of biofilm infections remain largely unknown because scientists currently have limited tools for producing biofilms with well-defined, user-tunable spatial structures. However, recently-developed methodological approaches have succeeded in producing structured biofilms on millimeter-length scales that are much larger than single bacteria or typical biofilm infections and in modifying surfaces thereby altering biofilm development’s natural course.
[adrotate group=”1″]
“We have devised a way to structure these bacterial systems with single-cell resolution while leaving unchanged the bacteria’s surface adhesion and motility,” say the coauthors. “To do this, we use a laser trap to place bacteria on a surface, one at a time, thereby building up arbitrary, user-defined, and reproducible configurations. A laser trap uses light focused through a high-magnification microscope objective to hold micron-sized objects with an index of refraction higher than that of the surrounding medium. When light transmits through a boundary between materials with different refractive indices, electromagnetic boundary conditions require that light not at normal incidence must alter direction, which changes the light’s momentum. Conservation of momentum requires that an equal and opposite change in momentum is imparted to the object in the trap, so that it is held near the focus of the laser beam.”
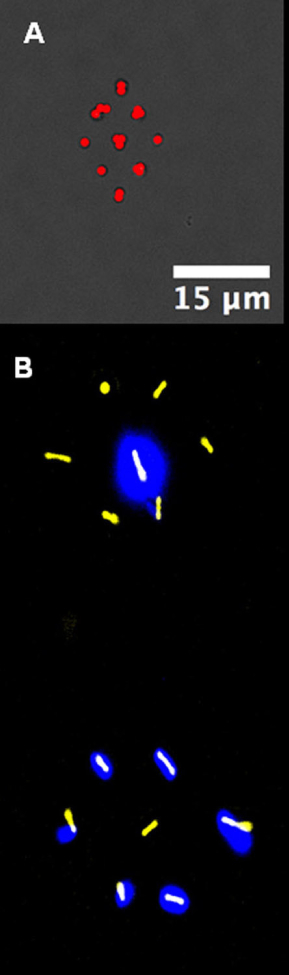
Image Caption – Patterns made up of single bacteria placed on a surface using a laser trap. (A) Staphylococcus (Staph) bacteria in a diamond. (B) Two strains of Pseudomonas bacteria, one non-fluorescent (false color yellow) and one fluorescent (false color halo glows), patterned in complementary hexagons.
The researchers add that bacteria typically measure about one micrometer (?m) in size and have a higher index of refraction in aqueous culture media, qualities that make ideal candidates for laser trapping in which lasers are employed to “trap” and manipulate electrically neutral particles. They note that intense interaction of laser radiation of with molecular oxygen can activate excited, highly reactive conditions that can damage or kill bacterial cells. They solved this problem by using an IR laser, minimizing levels of oxygen in the growth medium, and limiting the laser power and trap time duration. They surround their microscope stage (the platform on which trapping is performed) in an incubator enclosure in which temperature and humidity are precisely controlled in a light-tight environment, thereby enabling growth of biofilms under physiological conditions. Removable sections on the bottom of the microscope on both sides of the stage enable access to the objective turret and under-stage area, with the laser entering the microscope enclosure through a small hole through which it can be directed upward to the sample using a slider-controlled dichroic mirror.
Gordon and Rodesney investigated the opportunistic human pathogen Pseudomonas aeruginosa, which typically establishes biofilms in chronic wounds and in cystic fibrosis (CF) patients’ lungs, finding that small Pseudomonas clusters of fewer than 10 cells grown in a bovine albumin based medium containing (i.e.: a carbon source that breaks down by the action of extracellular proteases) develop approximately 15 percent faster than isolated clonal siblings do in the same medium.
The scientists observe that another pathogenic bacterium, Staphylococcus aureus (“Staph”), is a frequent co-pathogen with Pseudomonas, and that interspecies antagonism between the two bacteria strains can result in increased severity of Pseudomonas infections. However, they note that Pseudomonas and Staph biofilms are often small and isolated from direct contact with each other, even when present in the same infection site.
Consequently, they say it is beneficial to understand conditions required for Pseudomonas-Staph antagonism to be activated and to identify conditions which produce co-infections resulting in damaging outcomes. Gordon and Rodesney have taken the first step toward this by demonstrating that small clusters of 10 initial Pseudomonas cells reduce the Staph growth of by 10 percent.
In summary, the researchers say that in order to better understand how spatial arrangements in the microbial affect the aetiology of chronic biofilm infections, ways to control the structure of these biofilms on a single-cell level will be required, and that their work shows that this ca achieved for art least two disease-causing organisms – P. aeruginosa and S. aureus., and that the method they developed for P. aeruginosa is directly transferrable to S. aureus. Consequently, they think it likely that they’ve discovered experimental principles that can enable laser trapping to build structured biofilms across a wide spectrum of organisms.
Gordon and Rodesney acknowledge that laser trapping is not a tool commonly employed in microbiology departments, but the devices they use are commonplace in physics, chemistry, or engineering labs, and the other equipment used in their procedure is inexpensive and non-exotic, so the method they’ve developed could easily become a widely usable tool for microbiological and physical researchers in many types of scientific and clinical institutions. In their own investigations, the researchers say they intend to use the method they’ve developed to determine necessary conditions for S. aureus to stimulate virulence in P. aeruginosa infectons, in the context of both species’ spatial density and distribution, and transport conditions in particular environments.
Gordon and Rodesney’s research was supported by startup funds to author VDG from the University of Texas at Austin, a grant from the Human Frontiers Science Project, and Dr. Gordon thanks the Cystic Fibrosis Foundation for the postdoctoral fellowship she held that allowed her to develop much of this technique.
Dr. Vernita Gordon is an assistant professor of physics and a member of the Center for Nonlinear Dynamics and the Institute for Cellular and Molecular Biology. She likes finding new ways to use physics to understand and possibly remediate disease-causing biological systems.
Dr. Gordon’s group at UT Austin’s primary focus is on understanding interactions between two or more cells, and between cells and their environment — processes that are foundational to multicellular life — and especially especially how physics shapes these biological interactions and how physical tools and modes of thought can help scientists better understand them.
Chris Rodesney is a PhD candidate whose primary research focus is on the role of structure in early biofilm formation.
Sources:
SPIE
University of Texas at Austin
UTA Department of Physics’ Center for Nonlinear Dynamics
Image Credits:
SPIE
UTA Department of Physics’ Center for Nonlinear Dynamics