Can Gene Editing Techniques Like CRISPR-Cas9 Be Used to Replace Mutated Genes in CF?
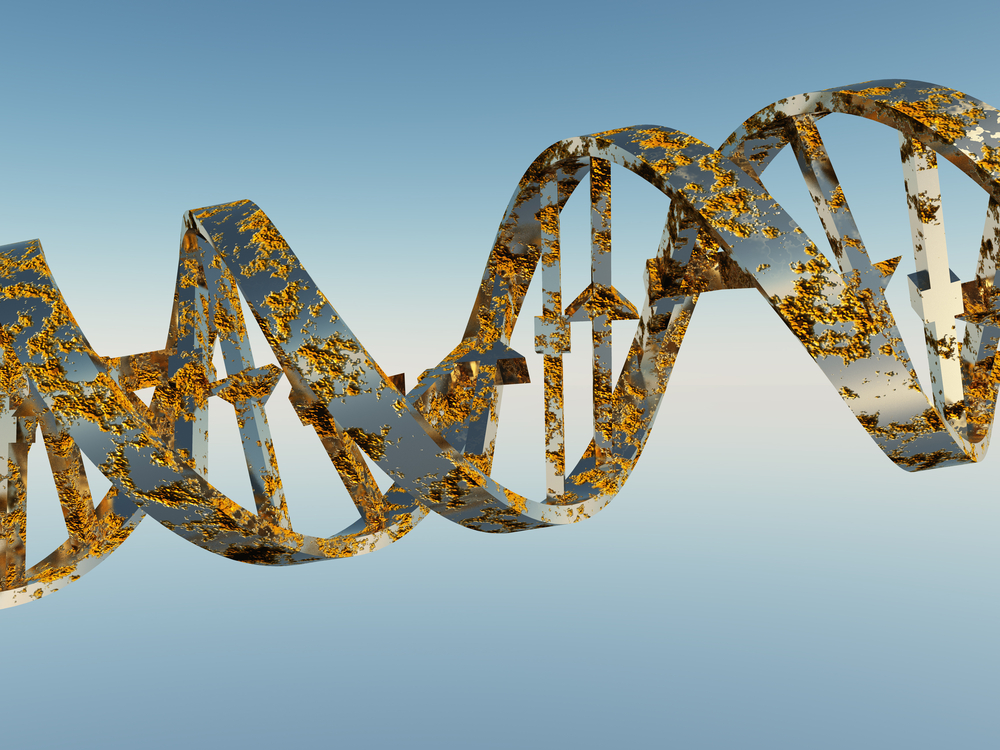
The FDA approval of the CFTR modulator, Kayldeco, in 2012 changed the paradigm of CF healthcare by providing a therapy to address the underlying cause of the disease. The previous paradigm in CF care was limited to only treat the symptoms of CF, such as inflammation, bacterial infection and mucous clearance. Although advancement within these ‘symptom-related’ therapies have done much to slow the progression of the disease and greatly extend the life expectancy of CF patients, they are incomplete solutions, as the outcome of the disease remains unaltered.
The shift that was introduced by Kayldeco opened the possibility that a patient was not resigned to only watch his or her health fail but that the potential existed that a patient’s health could begin to stabilize and even improve over time. Although the introduction of Kayldeco is not the final answer, it is the first stage in a progression of therapies that will lead the CF community to complete healing through a cure for the disease.
Research in gene editing has begun to demonstrate the possibility of replacing mutated genes through the use of CRISPR-Cas9, which uses a single stranded RNA to guide a nuclease to remove mutated genes in the genome. The CRISPR-Cas9 system holds potential in the development of gene therapies for genetic diseases like cystic fibrosis.
Briefly, cystic fibrosis is a genetically inherited disease that results from mutations in the cystic fibrosis transmembrane conductance regulator (CFTR) gene. The CFTR gene produces a protein in the cell that functions as a chloride channel in epithelial cells, which line passages in organs throughout the body. Mutations in this gene affect the protein by either reducing the quantity that makes it to the cell membrane, preventing the proper function of the protein on the cell membrane, or both. The resulting dysfunction creates dehydrated mucous in organs, specifically in the lung, which can lead to chronic lung infections, decreased lung function and end stage lung disease.
Therapies to address the underlying cause of CF can be directed to one of three levels in the cell: DNA, RNA, or protein. DNA, which is housed in the nucleus of the cell, contains the necessary instructions to create all the required proteins. The DNA, however, is not free to roam outside of the nucleus and, therefore, the instructions contained in DNA are transcribed or copied into RNA, which travels out of the nucleus where the directions are read to create the necessary protein. Proteins then are the final stage and are pivotal in providing the vital functions for maintaining cell survival.
[adrotate group=”1″]
Taken together, DNA is used to create RNA, which is used to create a protein – DNA -> RNA -> Protein. It then follows that if you have a mutation in your DNA, which is your primary level, there will also be a mutation in the RNA and a subsequent mutation in the protein.
In CF, mutation specific therapies can target the CFTR mutation at any of the three levels: DNA, RNA or protein. However, a cure can only be accomplished through repairing the mutation at the level of the DNA. CFTR modulators, like Kayldeco, which help to restore the function of the protein, do not address the RNA or DNA and, therefore, when more protein is synthesized by the cell it continues to be dysfunctional since the mutation persists in the DNA.
A cure and the end goal of CF therapies is to use gene editing to replace the mutated CFTR gene, which will restore normal function of the CFTR protein in patients. CRISPR-Cas9 has drawn considerable attention over the past few years because of its potential to be used in such a way to replace mutated genes in those with genetic disease.
The CRISPR-Cas9 system is able to target a specific sequence of DNA and cut it out, at which point the cell can fill in the excised portion with the correct gene sequence. Surprisingly, this system was discovered in bacteria as an adapted form of defense. Blake Weidenheft and colleagues in a Nature article in 2012 explain, ”in response to viral and plasmid challenges, bacteria and archaea integrate short fragments of foreign nucleic acid into the host chromosome at one end of a repetitive element known as CRISPR (clustered regularly interspaced short palindromic repeat). These repetitive loci serve as molecular ‘vaccination cards’ by maintaining a genetic record of prior encounters with foreign transgressors,” which are then used to identify and degrade the invading virus or DNA.
Prashant Mali and colleagues in a 2013 article published in Nature Methods provide an excellent review of the mechanism of the CRISPR-Cas9 system. The system operates by guiding Cas9, a nuclease which cuts DNA, to the site of gene excision by single stranded CRISPR RNAs (crRNAs). The excised DNA is then replaced by the cell’s DNA repair machinery by the use of either non-homologous end joining or homologous recombination.
The application of the CRISPR-Cas9 system has already begun to take shape in the arena of CF research. One study in 2013 published in Cell by Gerald Schwank and colleagues titled, Functional Repair of CFTR by CRISPR/Cas9 in Intestinal Organoids of Cystic Fibrosis Patients, used CRISPR to correct the CFTR gene in instentinal cells grown in culture.
In the study, small and large intestinal organoids were established from two pediatric donor patients, who were homozygous (two copies) for the F508del mutation. These intestinal organoids or “miniguts” are a brilliant culture system that allows cells to mimic the structure and function of intestines found within the body. The cultured organoids were shown to have dysfunctional CFTR function by use of a forskolin assay, measuring movement of fluid across the intestinal cells.
The question the study sought to answer was, is it possible to use the CRISPR-Cas9 system to replace a mutated CFTR gene with the correct gene sequence and thereby restore the function of the gene? The study, with use of the CRISPR system, was successful in replacing the mutated CFTR genes in the intestinal organoids and the replacement of the gene restored function of the CFTR as demonstrated by the forskolin assay demonstrating a proper flow of fluid across the intestinal cells.
The success of the study helps to demonstrate the potential use of CRISPR-Cas9 in the future to correct mutated CFTR genes in those living with cystic fibrosis. Although its potential is exciting, there are still challenges to address before this type of technology can be used in a clinical setting as a gene therapy for CF patients.
In his article, Prashant Mali outlines the hurdles that are necessary to be overcome. He points out that an efficient delivery system will have to be created that can effectively carry this large complex into cells within a living system, which is considerably more complicated than in a set of cells in a culture dish. Additionally, it is crucial to prevent off-target activity of the Cas9 nuclease, or the potential for it to cut the DNA in other parts of the genome other than the desired gene.
Although a considerable amount of time and work needs to be completed to refine the CRISPR-Cas9 system in repairing a mutated CFTR gene in a patient, it represents a small opening toward what could one day be a cure. I will wait with considerable anticipation, following the progression of this technology, hoping that it will find its way into a clinical setting.