Researchers ID Genetic Catalysts That Accelerate Evolution of Antibiotic Resistance
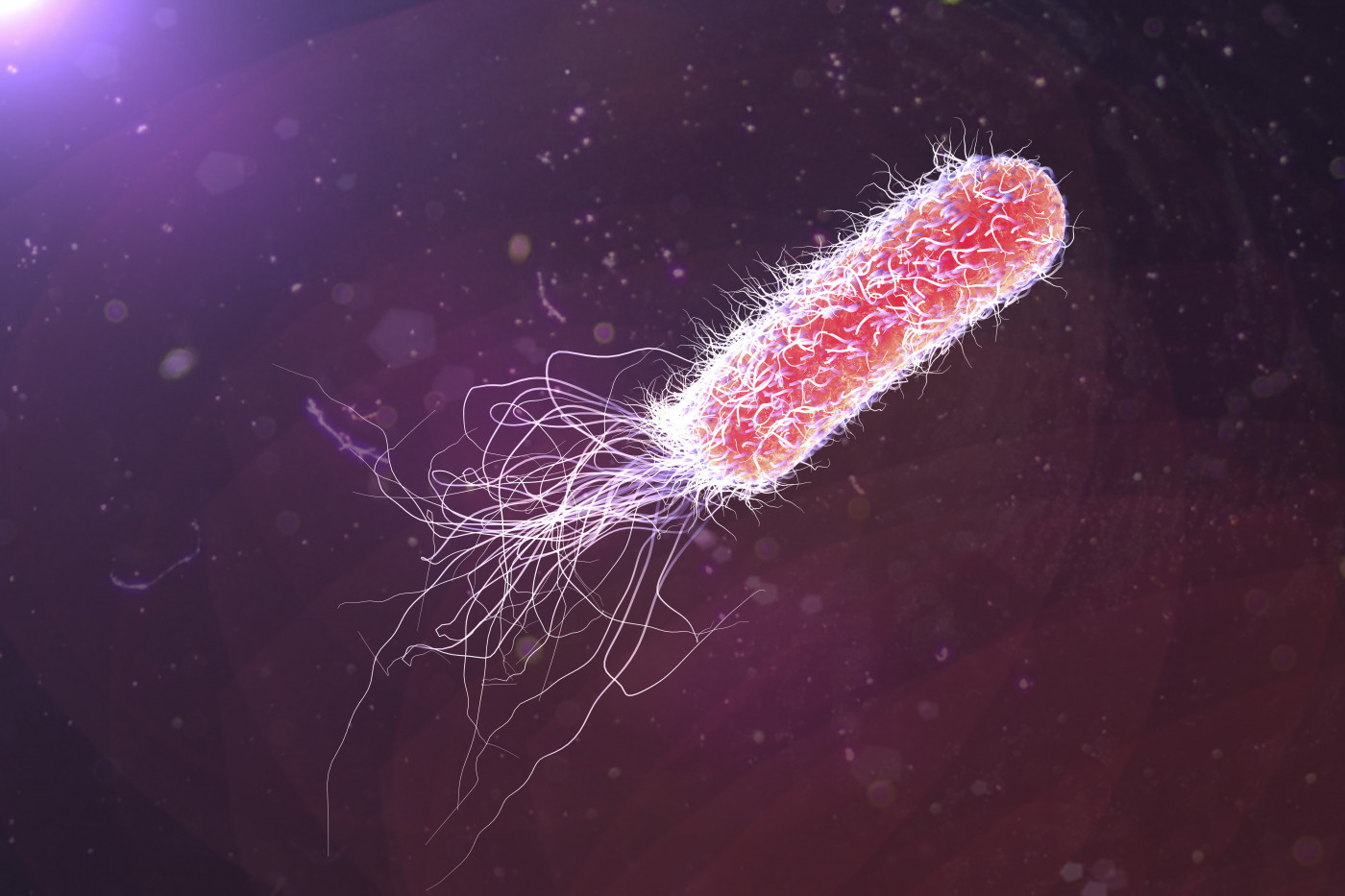
It is now possible to identify genetic catalysts that accelerate the evolution of antibiotic resistance in bacteria, thanks to a team of researchers at University of Oxford, U.K. The findings could be used to design treatments to stop the development of antibiotic resistance.
The data were published in the journal Nature Ecology & Evolution under the title “Identifying and exploiting genes that potentiate the evolution of antibiotic resistance.”
In the study, the team investigated the possibility that potentiator genes acted as catalysts promoting the evolution of antibiotic resistance. To test their hypothesis, researchers used species of Pseudomonas aeruginosa, a type of bacteria that can be life-threatening to several patients, including those with cystic fibrosis (CF).
They found that individual species of P. aeruginosa developed resistance against ceftazidime (a clinically important β-lactam antibiotic) at profoundly different rates.
“It is well established that different species of bacteria evolve resistance to antibiotics during infections at very different rates. One potential explanation for this observation is that some species of bacteria may be intrinsically better at evolving resistance to antibiotics than others,” Craig MacLean, the study’s senior author, said in a press release.
Researchers found this variation is linked to a gene called ampR (ampicillin resistance).
“ampR is a master regulatory gene that switches the expression of hundreds of other genes on and off, including genes involved in antibiotic resistance. This gene acts as an evolutionary catalyst for antibiotic resistance. Put simply, species that carry the ampR gene evolve resistance at a higher rate than species that lack this gene. ampR has this effect because it makes it easier for random mutations to increase the expression of antibiotic resistance genes,” MacLean said.
“Pseudomonas aeruginosa carries the ampR gene, and it evolved ceftazidime resistance at quite a high rate in our experiments. We therefore wondered if it would be possible in effect to remove the evolutionary advantage that this gene provides,” MacLean added.
The team used a combination of ceftazidime and avibactam (an inhibitor of a key resistance gene that is controlled by ampR) to treat cultures of P. aeruginosa bacteria. The experiment worked “spectacularly well,” the researchers said, eliminating populations of the bacteria before they could develop resistance.
The results suggest that besides being able to treat infections caused by resistant bacteria, avibactam can also be used to prevent the development of antibiotic resistance in the first place. The team is now aiming to conduct follow-up in vivo experiments to test their findings.
“This work has two important implications. First, our study shows how understanding the evolutionary biology of resistance can be used to come up with new ways to stop resistance from spreading. In this case, we show that using an enzyme inhibitor that blocks the evolutionary path to antibiotic resistance can be used to eliminate populations of an important pathogen without driving the spread of resistance. Second, this study shows that comparing how closely related species of bacteria become resistant to antibiotics can help us better understand resistance in pathogens,” MacLean said.
Antibiotic resistance is a growing public health concern. For instance, antibiotic-resistant infections are currently implicated in 700,000 deaths each year worldwide and are anticipated to surpass cancer as a source of mortality by 2050.
“Given this crisis, there is an urgent need to develop new ways of treating bacterial infections. The key idea that motivates our research is that we can use an understanding of how evolution works to come up with new ways of predicting and preventing the spread of resistance,” MacLean concluded.