Study delves into how CFTR changes its shape to be effective
Detailing link between protein's structure and function may advance treatment
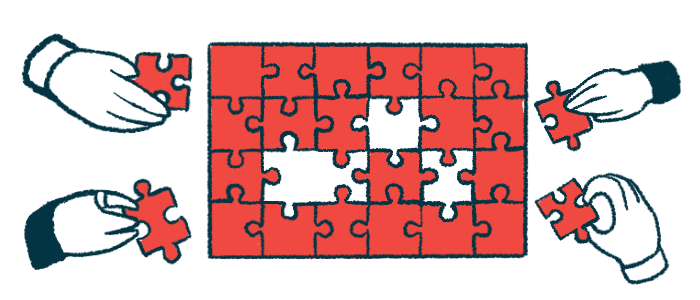
Small changes in the shape of CFTR, the protein defective or absent in people with cystic fibrosis (CF), opened its gate outside the cell to allow the flow of chloride ions, a study reported.
CF-causing mutations that suppress CFTR function disrupt these long-distance shape changes. By comparison, CFTR potentiator therapies, designed to open the gate, reinforce CFTR’s structure to support its functional movement.
According to the researchers, these discoveries could guide the development of new CF therapies that further enhance CFTR function.
“We have gotten to a unified mechanism that gives us insight into how this molecule works,” Jue Chen, PhD, the study’s co-lead scientist with Rockefeller University in New York, said in a press release. “With this understanding, we can then test how mutations or drugs affect the function, which is ultimately how we’ll get to better therapeutics.”
CFTR is a two-protein complex, each with three domains or parts
Details of the discovery were reported in “CFTR function, pathology and pharmacology at single-molecule resolution,” published in the journal Nature.
CFTR is a protein embedded in the cell membrane that transports negatively charged chloride ions in and out of cells. Chloride flow helps control the balance of water on tissue surfaces, ensuring that mucus lining the airways, digestive system, and other tissues remains free-flowing and protective.
In CF, inherited CFTR gene mutations result in a defective CFTR protein, which impairs chloride flow, alters water balance, and leads to the buildup of thick mucus and disease symptoms.
CFTR is a complex composed of two protein copies, each with three parts called domains. The transmembrane domains span the cell membrane to form the channel pore and gate, leading outside the cell. Inside the cell are the regulatory and nucleotide-binding domains (NBDs).
In response to signals within the cell, enzymes first modify CFTR’s regulatory domain by attaching a phosphate molecule (phosphorylation), which acts like an “on” switch. The channel opens when ATP, the primary carrier of energy in cells, binds to the NBDs and breaks down to ADP, releasing its energy to power the process.
Using a molecular imaging technique called cryo-electron microscopy, Chen’s team previously demonstrated that CFTR’s molecular structure formed two distinct shapes, each representing the open and closed forms. Before activation, the channel was closed and the two NBDs were far apart in space. With activation, the two NBDs came together — or dimerized — and the channel opened.
While these findings provided valuable insight into the relationship between CFTR’s structure and function, they could not explain how NBD dimerization triggered the opening of the CFTR channel.
Understanding how CF-causing mutations affect these processes, and how approved CFTR modulator therapies help correct channel defects, could support work into more effective treatments.
In this study, Chen collaborated with Scott Blanchard, PhD, at St. Jude Children’s Research Hospital in Tennessee, who specializes in single-molecule fluorescence resonance energy transfer (smFRET), a tool to monitor changes in protein shape in real time.
Together with Chen’s cryo-electron microscopy and electrophysiology methods measuring ion flow, the team investigated CFTR’s transition from closed to open states in detail.
How CFTR protein works topic of debate ‘for many years’
“Through this collaboration, we had the opportunity to really dial into the relationship between structure and function,” said Blanchard, the study’s other co-lead researcher. “There are very few single proteins that are more relevant for the treatment of disease than CFTR because treatments for cystic fibrosis are aimed at ameliorating the defects in the mutant forms of this protein.”
Researchers showed that ATP-dependent NBD dimerization alone was not enough to open the gate, as previously proposed. Rather, ATP’s breakdown altered the shape of the NBD-dimerized CFTR, twisting its shape on the opposite side of the membrane, opening the gate, and allowing chloride ions to flow.
“The most satisfying thing about this work is that we have answered a question about how CFTR works that has been a subject of debate in the field for many years,” Chen said. “Every individual method has limitations, so you can have good data but still not have the answers.”
Two CF-causing variants — G551D or L927P — are known as gating mutations because they block the CFTR channel from opening.
Data showed the G551D mutation affected the shape of the ATP binding site, which impacted NBD dimerization and disrupted the link between NBD and the gate. L927P is known to impair the transmembrane domain’s ability to mediate local shape changes during gating. Notably, this mutation also impaired NBD dimerization despite being located far from the NBDs in space.
Kalydeco (ivacaftor), an approved CF treatment, and the investigational therapy GLPG1837 are both potentiators, a class of CFTR modulators that work by binding to the transmembrane domain and holding the CFTR gate open.
Data showed that neither potentiator influenced NBD dimerization, or their coming together in space. Instead, they promoted gate opening when NBDs are already dimerized by enhancing the pathway linking the NBDs to the channel gate, “possibly by stabilizing the transmembrane domains in the pore-open configuration,” the researchers wrote.
“There is potential here to help cystic fibrosis patients by learning about the structure and behavior of CFTR,” said Jesper Levring, the study’s first author with Chen’s lab. “Looking at these molecules one at a time using these methods — single-channel electrophysiology and smFRET — we could correlate the function of the channel with the [shape] changes and relate it back to the underlying structural biology.”